What light from yonder neutron breaks?
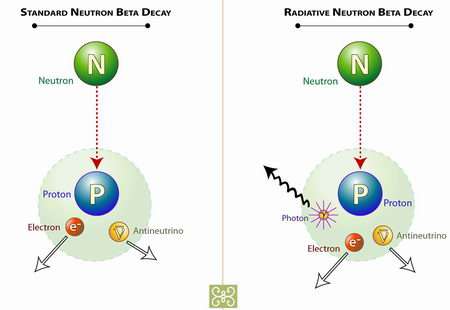
Researchers from the National Institute of Standards and Technology and four universities have made the first experimental observation of rare particles of light emitted during the radioactive decay of the neutron, a key building block of matter. This work confirms theoretical predictions of this type of decay of the neutron and sets the stage for a new class of tests of basic theories in particle physics.
The experiments, reported in the Dec. 21, 2006, issue of Nature, were performed at the NIST Center for Neutron Research (NCNR) in Gaithersburg, Md., because of the unique instruments and expertise at the facility. The authors include researchers from the NIST Physics Laboratory, Tulane University, the University of Michigan, the University of Maryland and the University of Sussex (Brighton, England).
The team determined that slightly more than three out of 1,000 neutron decays on average (3.13 ± 0.34 x 10^-3 to be precise), produce a photon (a particle of light) above an energy level that is relatively low but still observable. The measured value has only about 10 percent uncertainty, which is considered remarkable given that this decay had never been observed before.
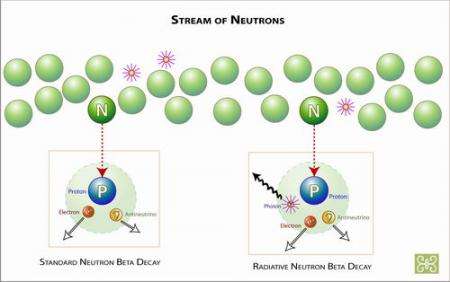
"This measurement is difficult because the neutron lifetime is very long, so very few neutrons decay at one time, and of those that decay, very few emit a photon," says NIST physicist Jeffrey Nico, lead author of the paper. "To make it even worse, the background radiation is very large."
The neutron is stable only when confined in the nucleus of a stable atom; a free neutron decays into other particles--a proton, electron and anti-neutrino--within about 15 minutes. This process, known as "neutron beta-decay," has been studied for decades without proof of the occasional photon emissions predicted by theory. These photons can be easily missed amid the intense background radiation associated with a neutron beam. The researchers adapted existing instruments and techniques to minimize uncertainties and unwanted background effects, and designed a clever experiment to prove that they had indeed found the elusive photons. They also used a novel hybrid photon detector that could operate in the high magnetic field and at the extremely cold temperatures of the experiment.
The experiments, conducted from January 2004 to November 2005, used neutrons chilled to very low ("cryogenic") temperatures which slowed them down for easier detection. A stream of neutrons was directed into a chamber immersed in a strong magnetic field. When a neutron decayed, the magnetic field transported the resulting charged decay products that were emitted backwards (electrons with a negative charge and protons with a positive charge) toward a silicon detector. The neutral photons continue toward the hybrid detector (a combination of a special crystal and a photodiode).
Each electron reached the particle detector several microseconds before its slower counterpart proton from the same neutron. The researchers looked for only those photons that arrived at the crystal simultaneously with an electron and were followed after a specific time by a proton--and thus could be expected to derive from the same neutron.
A key tool in this experiment was an electrostatic mirror electrode, near the photodiode, that could be manipulated to reflect stray protons back to the detector. The researchers found the signature of photons from neutron decay by varying the voltage applied to the mirror, thus altering the number and energy of protons reflected back to the detector. This changed the rate of electron-proton coincidences, as well as the rate of correlated photons, without affecting the rate of background photons arriving at the detector. This enabled scientists to separate the two groups of photons. The data collected with various mirror settings agreed with theoretical predictions.
This successful experiment now makes it possible to design new tests of the "standard model" of particle physics and search for new physics beyond it, according to the paper. With future upgrades to the experimental set-up, the authors say they could enhance measurement precision further and open up additional opportunities for pushing the boundaries of scientific knowledge.
Citation: J.S. Nico, M.S. Dewey, T.R. Gentile, H.P. Mumm, A.K. Thompson, B.M. Fisher, I. Kremsky, F.E. Wietfeldt, T.E. Chupp, R.L. Cooper, E.J. Beise, K.G. Kiriluk, J. Byrne and K.J. Coakley. Observation of the radiative decay mode of the free neutron. Nature (Dec. 21, 2006).
Source: National Institute of Standards and Technology